Harnessing Bioengineering: The Future of Health and Innovation
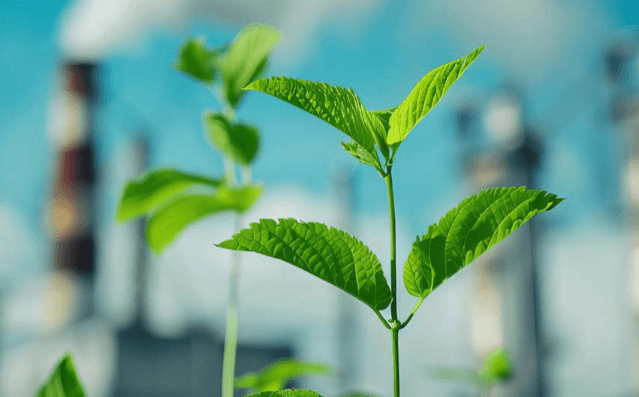
In an age marked by rapid technological advances, bioengineering stands at the intersection of biology and engineering, paving the way for revolutionary changes across various industries. This multidisciplinary field is not only driving innovation in healthcare but is also transforming agriculture, energy, and environmental sustainability. By applying engineering principles to biological systems, bioengineering offers promising solutions to some of the most pressing global challenges. From personalized medicine to synthetic biology and tissue engineering, bioengineering is reshaping our future.
The Foundations of Bioengineering
Bioengineering, also known as biological engineering, involves the integration of biology with principles of engineering to develop new technologies and systems that address biological problems. This field spans numerous sub-disciplines such as biomedical engineering, genetic engineering, and tissue engineering, among others. It uses techniques from mathematics, physics, chemistry, and computer science to understand complex biological processes, create innovative medical devices, and develop novel solutions to environmental issues.
The role of bioengineering extends far beyond healthcare. Bioengineers are instrumental in developing new agricultural techniques to improve food production, designing biocompatible materials for medical implants, and creating renewable energy solutions through biofuels. With the rise of artificial intelligence (AI) and machine learning, bioengineering is becoming even more powerful, as these tools allow for the analysis of vast datasets and optimization of complex biological systems.
Personalized Medicine: Tailoring Treatments to Individuals
One of the most exciting applications of bioengineering is in personalized medicine. Traditional medicine often takes a one-size-fits-all approach, but bioengineering is enabling the development of treatments that are tailored to individual patients. By analyzing a patient’s genetic makeup, bioengineers can design targeted therapies that are more effective and have fewer side effects.
For example, cancer treatment is being revolutionized by personalized approaches. Tumors have unique genetic mutations, and by understanding these, bioengineers can develop therapies that specifically target the cancer cells, leaving healthy cells unharmed. This precision medicine approach is already showing promising results in treating cancers like melanoma and breast cancer.
The rise of CRISPR gene-editing technology has further pushed the boundaries of personalized medicine. CRISPR allows scientists to make precise changes to DNA, opening the door to treating genetic disorders at their source. Diseases like sickle cell anemia and cystic fibrosis, once thought incurable, may now be treatable through gene editing. This technology represents a major leap forward in personalized medicine, as it allows for interventions that are customized to an individual’s genetic profile.
The Development of Artificial Organs and Tissues
Bioengineering is playing a critical role in addressing the shortage of donor organs. Every year, thousands of patients die waiting for organ transplants, but bioengineers are working on solutions that could eliminate this problem. Through advancements in tissue engineering and 3D bioprinting, scientists are developing artificial organs that could one day replace damaged or failing organs in humans.
The creation of lab-grown tissues has already seen significant progress. Researchers have successfully developed lab-grown skin, blood vessels, and cartilage, which are being tested for use in human patients. The hope is that fully functional artificial organs—such as hearts, kidneys, and livers—could be created from a patient’s own cells, minimizing the risk of rejection and offering a sustainable solution to organ shortages.
One particularly promising technique is 3D bioprinting, where bioengineers use specialized printers to create layers of living cells that form tissues and, potentially, entire organs. Although creating a fully functioning organ is still in the experimental stages, bioengineers are optimistic that these technologies will soon be able to produce organs that can be implanted in human patients.
Genetic Engineering: Transforming Agriculture and Healthcare
Genetic engineering, a core component of bioengineering, involves altering the DNA of living organisms to introduce new traits or eliminate harmful ones. This technique has broad applications in both agriculture and medicine, making it a powerful tool for innovation.
In agriculture, genetic engineering is being used to create crops that are more resistant to pests, diseases, and environmental stresses. These genetically modified crops can grow in harsh conditions, helping to ensure food security in regions affected by climate change. Additionally, bioengineers are developing crops with enhanced nutritional profiles to address malnutrition and vitamin deficiencies in vulnerable populations.
In healthcare, genetic engineering offers the potential to cure genetic diseases. By editing faulty genes that cause hereditary conditions, such as Huntington’s disease or muscular dystrophy, scientists hope to develop treatments that can correct these genetic errors at the source. Gene therapies, where corrected genes are delivered to a patient’s cells, are already showing promise in clinical trials. For example, gene therapies for conditions like hemophilia and leukemia are currently being tested, offering hope to millions of patients.
Synthetic Biology: Engineering Life from the Ground Up
Synthetic biology is a groundbreaking sub-discipline within bioengineering that involves designing and constructing new biological systems from scratch. By reprogramming living cells or even building entirely new organisms, synthetic biologists are unlocking new possibilities in fields ranging from healthcare to energy production.
One of the most exciting applications of synthetic biology is in the development of biofuels. Scientists are engineering microbes that can convert renewable resources, such as plant waste or algae, into biofuels. This approach offers a cleaner, more sustainable alternative to fossil fuels and could play a significant role in reducing our reliance on nonrenewable energy sources.
In medicine, synthetic biology is being used to design cells that can detect and respond to diseases in the body. For instance, bioengineers are developing “smart” cells that can seek out and destroy cancer cells without harming healthy tissue. These engineered cells could serve as living drugs, providing a highly targeted approach to treatment.
Advancing Environmental Sustainability
Beyond healthcare, bioengineering is contributing to environmental sustainability by developing new methods for cleaning up pollution and managing natural resources. One of the most promising techniques in this area is bioremediation, where engineered microbes are used to break down toxic substances, such as oil spills or hazardous chemicals, into less harmful byproducts.
For example, bioengineers are developing bacteria that can metabolize plastics, offering a potential solution to the global plastic waste crisis. These engineered organisms can break down plastic materials much more efficiently than current methods, reducing the environmental impact of plastic pollution.
Additionally, bioengineering is playing a key role in the development of sustainable agricultural practices. Precision agriculture, which uses bioengineering tools to monitor and manage crops more efficiently, allows farmers to reduce the use of chemical fertilizers and pesticides. This not only lowers costs but also minimizes the environmental damage caused by agricultural runoff.
Drug Development and Delivery Systems
Drug development is an expensive and time-consuming process, but bioengineering is helping to streamline this by using advanced technologies to predict how new drugs will interact with the human body. By using computational models and simulations, bioengineers can test the effects of potential drugs before they are introduced into clinical trials, reducing both the cost and time involved in developing new treatments.
Moreover, bioengineering is revolutionizing drug delivery systems. Nanotechnology, a growing field within bioengineering, allows scientists to design nanoparticles that can deliver drugs directly to specific tissues in the body. This targeted delivery method minimizes side effects and increases the effectiveness of treatments, especially in cases like cancer, where traditional chemotherapy can harm healthy cells.
Regenerative Medicine: Repairing and Replacing Human Tissue
Regenerative medicine, which focuses on repairing or replacing damaged tissues and organs, is one of the most exciting fields in bioengineering. Stem cells, which have the ability to develop into any type of cell in the body, play a central role in regenerative medicine. Bioengineers are exploring how to use stem cells to treat conditions like spinal cord injuries, heart disease, and neurodegenerative disorders.
One promising application of regenerative medicine is in the treatment of degenerative diseases, such as Parkinson’s disease and Alzheimer’s. By using stem cells to replace damaged or dying cells in the brain, researchers hope to restore lost function and improve the quality of life for patients suffering from these conditions.
Additionally, bioengineers are developing new biomaterials that can be implanted in the body to promote tissue regeneration. These materials provide a scaffold that supports the growth of new cells and tissues, helping the body heal from injuries that would otherwise be irreversible.
The Ethical Implications of Bioengineering
While bioengineering offers tremendous potential for innovation, it also raises important ethical questions. One of the most debated topics is the use of genetic engineering in humans. The possibility of editing the human genome to prevent diseases or enhance certain traits has led to concerns about the potential for misuse, including the creation of “designer babies” where parents select the traits of their children.
Another ethical issue involves the use of animals in bioengineering research. Animal testing has been instrumental in many medical breakthroughs, but it also raises concerns about animal welfare. In response, bioengineers are working on alternatives, such as organ-on-a-chip technology, which mimics the functions of human organs in the lab and reduces the need for animal testing.
As bioengineering continues to advance, it will be crucial to develop ethical guidelines that balance the potential benefits of these technologies with the need to protect human rights and prevent misuse.